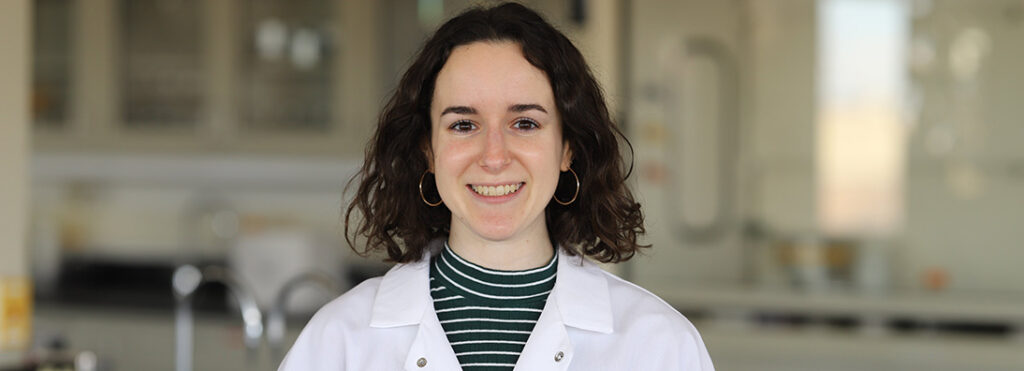
Biomedical and chemical engineering graduate student Natalie Petryk ’21, G ’22 won first place in the student pitch competition at the BioInterface Symposia 2021. Petryk is a part of biomedical and chemical engineering Professor Mary Beth Monroe’s research team and submitted a short pitch on tuning pore structure of polyurethane shape memory polymer (SMP) foams using EPA recommended foaming agents and characterizing the effects of pore structure on blood and cell interactions.
Below is a summary of her experience at the BioInterface Symposia, the pitch competition and her research.
“Over two years ago, I started working in Dr. Mary Beth Monroe’s lab as an undergraduate student simply wanting to gain more research experience. Never did I think my research in her lab would translate into the love and passion for biomaterials-related research that I have now. Now, as a Bioengineering M.S. student in the Monroe Biomaterials Lab, I have been able to further explore my research interests and build upon exciting work that I was fortunate to present in the BioInterface Student Pitch Competition.
Having the opportunity to share my current research as part of the BioInterface Student Pitch Competition was a fun, invaluable experience that I am grateful to have taken part in. It was fascinating to learn more about advances being made in biomaterials research and industry from both students and professionals in the field. Presenting my research in the form of a pitch truly allowed me to reflect on the significance of my work, because I was focused on conveying its potential impact to a broader audience. Overall, attending the BioInterface Workshop and delivering my pitch confirmed my interest in biomaterials and my future career goals.
Polyurethane shape memory polymer (SMP) foams are “smart” materials that can switch between a primary and secondary, deformed shape when exposed to an external stimulus.[1] This behavior allows SMP foams to have wide-ranging biomedical applications in areas such as drug delivery, tissue engineering, and wound healing; in these applications, tuning the pore structure of SMP foams can greatly affect drug release rate, cell proliferation and migration, and hemorrhage control, respectively.[2]–[5] The ease of tuning both pore size and interconnectivity could greatly aid in future commercialization efforts of SMP foams for multiple uses. My work explored off-the-shelf solvents and their use as physical blowing agents to safely and easily tune pore structure.
Physical blowing agents are incorporated during foam synthesis, and unlike chemical blowing agents, they do not affect foam chemistry; they boil off during foaming, forming bubbles that result in pores throughout the polymer.[6] Enovate is a physical blowing agent commonly used in gas-blown foaming, but the Environmental Protection Agency (EPA) considers its use unacceptable because it is a hydrofluorocarbon that can contribute to global warming.[7] It also comes from a single supplier, making commercial use risky.
We selected three physical blowing agents that are both commercially available and recommended for use by the EPA in their Significant New Alternatives Policy (SNAP) Program: acetone, dimethoxymethane (methylal), and methyl formate.[8] We found that increasing the volume of solvent added during foam synthesis (1 mL, 2 mL, and 3 mL) increased the interconnectivity between pores while maintaining chemical and thermal properties of the SMP system. This work provides a safe and easy method to create a more open, porous structure that could be tailored for different applications.
More recently, I explored the effects of tuning foam pore structure on blood and cell interactions with foams synthesized with methyl formate. We found that foams with more interconnects (i.e., synthesized with a higher volume of methyl formate) had thrombus formation after 1 hour of incubation in anticoagulated blood, which suggests that the clotting ability of these foams could be harnessed in traumatic wound healing applications. We also saw that larger pore size and fewer interconnects among control foams and foams synthesized with 1 mL methyl formate resulted in higher cell attachment. This result could be an effect of the higher overall surface area that cells could attach to, an important consideration for designing tissue engineering scaffolds.
Future work involves synthesizing a completely off-the-shelf foam to make the commercialization of polyurethane SMP foams more feasible. This research will require substituting our current single-supplier catalysts with commercially available options, as well as synthesizing our own surfactant with off-the-shelf components. I also hope to look at blood perfusion through foams with different levels of interconnectivity and eventually explore vascularization as a function of foam interconnectivity.
My work in the Monroe Biomaterials Lab has opened my eyes to the potential of biomaterials. I am extremely thankful for Dr. Monroe’s support and guidance over the last 2 and half years, as my research under her has shaped my future career goals; after completing my master’s, I plan to pursue a Ph.D. and eventually work in industry doing biomaterials R&D.
I would also like to thank and acknowledge Grace Haas and Anand Vakil for their help with this project. Lastly, I am thankful for opportunities like the BioInterface Student Pitch Competition to be able to share my work with broader audiences that are also passionate about biomaterials.“
References:
[1] H. Y. Jiang, S. Kelch, and A. Lendlein, “Polymers move in response to light,” Adv. Mater., vol. 18, no. 11, pp. 1471–1475, Jun. 2006, doi: 10.1002/adma.200502266.
[2] U. T. Gunathilake Sampath, Y. Chee Ching, C. Hock Chuah, J. J. Sabariah, P.-C. Lin, and R. Luque Alvarez de Sotomayor, “materials Fabrication of Porous Materials from Natural/Synthetic Biopolymers and Their Composites,” doi: 10.3390/ma9120991.
[3] G. Lemon, Y. Reinwald, L. J. White, S. M. Howdle, K. M. Shakesheff, and J. R. King, “Interconnectivity analysis of supercritical CO2-foamed scaffolds,” Comput. Methods Programs Biomed., vol. 106, no. 3, pp. 139–149, 2012, doi: 10.1016/j.cmpb.2010.08.010.
[4] B. Otsuki, M. Takemoto, S. Fujibayashi, M. Neo, T. Kokubo, and T. Nakamura, “Pore throat size and connectivity determine bone and tissue ingrowth into porous implants: Three-dimensional micro-CT based structural analyses of porous bioactive titanium implants,” Biomaterials, vol. 27, no. 35, pp. 5892–5900, Dec. 2006, doi: 10.1016/J.BIOMATERIALS.2006.08.013.
[5] “BMP‐Induced osteogenesis on the surface of hydroxyapatite with geometrically feasible and nonfeasible structures: Topology of osteogenesis.” https://onlinelibrary.wiley.com/doi/epdf/10.1002/%28SICI%291097-4636%28199802%2939%3A2%3C190%3A%3AAID-JBM4%3E3.0.CO%3B2-K (accessed Sep. 01, 2021).
[6] K. H. Choe, D. L. Soo, W. J. Seo, and W. N. Kim, “Properties of rigid polyurethane foams with blowing agents and catalysts,” Polym. J., vol. 36, no. 5, pp. 368–373, 2004, doi: 10.1295/polymj.36.368.
[7] W.-T. Tsai, “An overview of environmental hazards and exposure risk of hydrofluorocarbons (HFCs),” Chemosphere, vol. 61, no. 11, pp. 1539–1547, 2005, doi: https://doi.org/10.1016/j.chemosphere.2005.03.084.
[8] “Substitutes in Flexible Polyurethane | US EPA.” https://www.epa.gov/snap/substitutes-flexible-polyurethane.